Is Origami the Future of Tech?
Nature uses folding to manufacture some of its most intricate creations, from flowers and wings to protein and DNA. What if humans could do the same?
In 1996 a young mathematician and computer scientist named Erik Demaine became fascinated by a magic trick that Harry Houdini used to do before he made his name as an escape artist. The magician would fold a piece of paper flat a few times, make one straight cut with a pair of scissors, and then unfold the paper to reveal a five-pointed star. Other magicians built on Houdini’s fold-and-cut method over the years, creating more intricate shapes: a single letter, for example, or a chain of stars. It’s an odd subject of study for a computer science professor, but Demaine had an unorthodox background. When he was hired by the Massachusetts Institute of Technology in 2001, he was, at 20, the youngest professor in the university’s history. Pale, thin, and soft-spoken, with a pickpocket’s long fingers and a fox-colored ponytail, Demaine was born in Halifax, Nova Scotia, and raised by his father, Martin, a renowned glass blower.
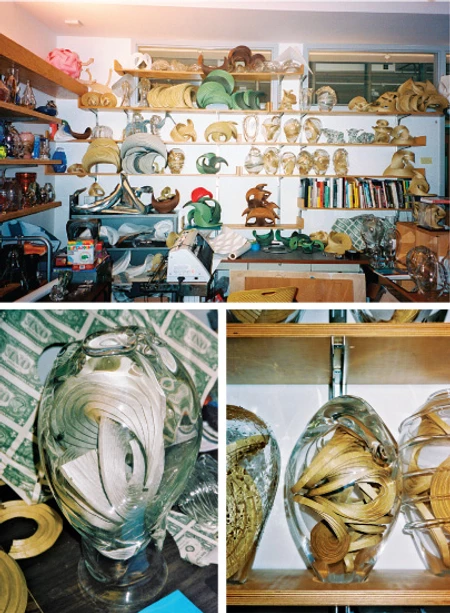
When Demaine was six, he and his father started a puzzle company. When he was seven, his father took him out of school and they spent four years traveling the U.S., choosing their destinations together. They spent a few months in Florida, Demaine recalls, “because it was so flat and good for riding bicycles.” Martin home-schooled his son while they moved from place to place. The traveling stopped when Erik returned to Halifax to enroll in Dalhousie University at age 12. It was his father who brought the fold-and-cut problem to his attention when, still a teenager, Demaine was searching for a dissertation topic. It was clear that one could create complicated shapes by folding and cutting, but how complicated? What were the limits? It was a simple but potentially universal question, exactly the sort that most appealed to Erik. He worked on the problem for two years and in 1998 published a paper with his answer: There were no limits. Fold-and-cut allowed you to make any shape in the world, any collection of shapes, even, as long as they had straight sides. One could, in an angular font, create the entire text of this page with the right folds and the right cut. In a paper published two years later, Demaine expanded on this idea, extending it into three dimensions: Any faceted solid, he showed, no matter how complex or irregular, could be folded from a single uncut sheet of paper. Start with a piece of paper big enough, and you could model Notre Dame down to the last gargoyle. At least in theory. Demaine’s computational origami work assumed an idealized sheet of paper with a thickness of zero, a sheet that could be folded an unlimited number of times. Still, his method gave a sense of the possibilities of folding. He has since become an accomplished origami artist. He’s also made a robot from a single sheet of plastic. When we think of mass production, the image is of a factory floor. Take a car. The engine block is cast, either from iron or aluminum. The hood, doors, and roof are stamped out on 100-ton presses. Gears are carved from metal blocks by milling machines or punched out by dies. The console and interior handles are injection-molded or carved, the mats and seats woven or stitched together. Some of these processes date to the Industrial Revolution, others to the Iron Age. The natural world doesn’t use any of them. One of its favorite methods is to take something flat and fold it into a three-dimensional form. Flowers, leaves, wings, proteins, mountain ranges, eyelids, ears, DNA—all are created by folding.
Today researchers in robotics, biology, math, and computer science are immersing themselves in that method. Scientists are looking at how materials and molecules wrinkle, drape, flex, and crease. They’re using folding to design everything from robots to cancer drugs, from airbags to mirrors for satellite telescopes. An Oxford University engineer named Zhong You has used origami to design better-crumpling car bumpers and flexible, low-cost stents. A team at Wake Forest University has used origami folding to create a fabric of densely layered nanotubes that can generate power from body heat. In a range of fields, fabrication by folding has the potential to be far faster, cheaper, and less energy-intensive than traditional methods and to work at very, very small scales, where even the most precise mills and lathes have all the accuracy of an earthquake. Makers of medical equipment and consumer electronics are looking at folding as a way to streamline manufacturing processes. “We have a paradigm where we want to build things by having a solid block and then etching away at the block until you get whatever shape that you want,” says William Shih, a Harvard University biochemistry professor. Think of Michelangelo chiseling his forms from boulders of marble, or a milling machine carving an engine part out of a hunk of steel. “The way that nature does things is different,” Shih says. “It uses a folding algorithm, and it’s something that seems to be very efficient. We can look to nature for inspiration.” Shih himself has designed devices at the nanoscale that assemble themselves out of DNA strands, a process known as DNA origami.
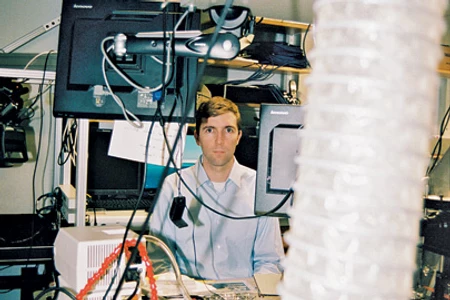
Folding is, at heart, a geometry problem, and the groundwork for much of the new research is being laid by mathematicians. The increasingly ingenious applications, though, are driven by collaborations between engineers, scientists, and programmers: “Biologically inspired engineering” is an ambitious new way of doing science that treats living organisms like mechanical systems. Just as the diameter of a gear or the strength of a spring determines how a clock works, the shape and tensile qualities of folded proteins determine their roles in the countless processes that keep the human body running. Deciphering those relationships and building off of them are part of what the new science of folding is about. Two of Demaine’s collaborators are the roboticists Robert Wood of Harvard and Daniela Rus of MIT. Rus is one of the world’s leading researchers of “programmable matter”: substances that can change their own physical properties. Programmable materials could allow for the creation of devices that take on multiple dissimilar tasks, repair themselves, or evolve. The Defense Advanced Research Projects Agency, or Darpa, has a programmable matter project, and it helped fund the work that Wood, Rus, and Demaine have done together. Most programmable matter research has focused on small modular robots and other devices that can combine into various larger forms. Folding is a different approach, and it has the advantage of having been studied both by mathematicians such as Demaine and by generations of origami practitioners. As Rus, Wood, and Demaine wrote in a June 2010 paper in the Proceedings of the National Academy of Sciences, programmable matter that folds could be used to create a Swiss Army knife-like tool that could morph from a wrench to an antenna to a tripod. Rus has spoken of the possibility of kinetic maps that could reproduce the topography of the landscape they’re showing, and Demaine of solar panels reconfiguring themselves to more efficiently capture sunlight.
The first challenge to realizing such machines is figuring out whether the sort of folding required is conceptually possible. Origami is all about crease patterns: choosing a fold pattern locks one into a single final form, whether it’s a crane or a flower. Demaine wanted to find out whether there was a crease pattern that was an exception to this, a sort of universal origami building block. He discovered it in a fold called the box pleat: a square grid of creases with alternating diagonals that origami artists had long known could be used to create a wide variety of different shapes. Demaine’s contribution, once again, was to prove just how wide that variety was. Using computational geometry, he proved that the box pleat worked as a three-dimensional pixel (a voxel). “If you want to make any shape out of little cubes, then this crease pattern is enough,” he says. Geometrically speaking, all you had to do was start with enough squares. In the summer of 2008, Rus, Wood, and the researchers in their labs set out to see if Demaine’s mathematical proof could be translated into an actual device. Their aim was a small prototype, a sheet that could fold itself into two simple shapes: an airplane and a boat. Paper thickness and paper size, the issues that always separate computational origami from real world folding, would only be exacerbated in this transformer robot. The “paper” would need circuitry, and the wires would have to be able to stretch at the robot’s joints, since each fold doubled the sheet’s thickness. In addition, the motors at the folds that would drive the transforming process would have to be very thin but also powerful, since they would be acting at the joint, the point of least leverage (think of pushing open a door right next to the hinge rather than at the side where the handle is).
Over the next year the designers came up with solutions to each of the challenges. The final sheet, a square half a millimeter thick and 4 centimeters to a side, comprises 32 identical fiberglass triangles with silicone rubber joints between them. To create the wiring for the joints, Rus and Wood cut copper-laminated plastic into a mesh so it could expand, accordion-like, across the folds while still carrying a current. The robot’s muscles were thin foil strips made of a “shape memory” metal alloy that would either fold or straighten when electricity was run through it. Its robot brain was a sticker. Since his days as a University of California at Berkeley graduate student, Wood has been working on developing a life-size robotic bee. A tiny aerial robot, he argues, could be used to gather information in military and search-and-rescue operations, to explore hazardous environments, even to pollinate plants if bee populations continue to decline. Today, Wood’s RoboBee is part of an arms race of small drones: There’s also the Nano Hummingbird, built by AeroVironment for Darpa, and the 22-inch quadrotors being developed at the University of Pennsylvania (their feats of coordinated flying have made them YouTube sensations). The RoboBee remains a more rudimentary flyer; its designers are still working on getting it into a stable hover. The considerable challenge of sustained, controlled flight is exacerbated for the RoboBee by the fact that it’s a fraction of the size of its competitors—39 mm from wingtip to wingtip, 18 mm from head to tail, and one-sixtieth the weight of a quarter. The RoboBee’s miniature scale has also created fabrication problems. Until recently the robots had to be built by hand using microscopes, tweezers, and superglue. The laborious process took a year to learn, and even then the merest twitch of an assembler’s fingers could ruin the work. Nine out of 10 parts ended up defective. At Harvard, Wood and the graduate students in his lab were constantly looking for ways to improve the process. A couple of them, including Wood, have small children, and two years ago they realized they had a model for how to rethink their fabrication process right in their homes: pop-up books. “You open up the page, and out pops this complicated structure,” Wood says. “All of the assembly trajectories are built into that laminated two-dimensional structure.” The magic of pop-up is that the clumsiest infant can make it happen. The unfolding is easy; what’s tricky is making the cuts and folds beforehand that ensure that everything properly deploys. If the engineers in the lab could create an assembly method that did the same thing, they would be able to turn a painstaking task into a mass production process. The minirobots could be stamped out by the sheet. Two of Wood’s graduate students, Pratheev Sreetharan and J. Peter Whitney, set to work on pop-up assembly, poring over how-to books on pop-up design and trading e-mails with a German pop-up sculptor. Sreetharan, a physicist by training, took the lead in creating a production process for the RoboBee. He had to map out the dizzying choreography that would, in a single movement, lift every piece of the bee from two dimensions into three, without any of the parts colliding as they swung into place. He spent months on a computer design program diagramming the cuts he would have to make to guarantee the right folds, and he built large-scale models in cardboard and glue to test his ideas. “One of the strengths I brought to this,” says Sreetharan, “was the fortitude to just spend that long working on it.”
Wood is one of 17 founding faculty members of an ambitious research alliance at Harvard called the Wyss Institute, whose mission in large part is to work with researchers to commercialize their ideas. What particularly excites him and his colleagues about pop-up folding is that it potentially can be used to make all sorts of complex devices. “It’s a manufacturing strategy that we think is going to revolutionize everything from microelectronics to toy manufacturing,” says Donald Ingber, a Harvard Medical School professor and director of the Wyss.
One of the companies working with Wood’s group is the Hong Kong-based toymaker WowWee, whose past hits include the Robosapien toy robot and Paper Jamz, a set of musical instruments made out of circuit-embedded paper. “We’re very interested, ” says Davin Sufer, the company’s chief technology officer. “We have a few product concepts that we’re working on together right now.” WowWee is looking at using pop-up fabrication to make toys that, like the RoboBee, would combine complex electronics with precision moving parts. “Toys are typically very labor intensive; we can save costs and make products more efficiently this way,” Sufer says. “We’re also looking at making products smaller and more compact than we could otherwise.”
The basic concept was to stack all of the bee’s building materials—multiple layers of carbon fiber (for the body), titanium (the wing frames), piezoelectric ceramic (to flap the wings), and a flexible plastic polyimide film (the joints)—one on top of the other like plywood, using dowels to align them. Each of the 18 strata was precision-cut with a laser, about 3,000 cuts per layer, and bonded to its neighbors at particular points with a solid adhesive. Some of the robot’s structural elements, such as the wing frames, were made from just one layer. Others emerged from the interaction of multiple strata—the joints, for example, are polyimide sandwiched between layers of carbon fiber, with small gaps cut out of the stiff carbon to allow for articulation, like the elbows in a suit of armor. The circuitry for sensing and controlling the RoboBee’s flight, Sreetharan says, can simply be printed onto some of the layers with the same techniques chip companies use to make circuit boards. By the spring of last year, Sreetharan thought he was close. He had come up with the idea of laser-cutting the carbon fiber around the bee into what he called an “assembly scaffold,” an intricate network of joints that would guide each component in the stack to its place as it rose, then could be easily cut away once the bee was locked into shape. One day in April, he laser-cut a prototype and, placing it under a microscope, began very slowly to raise it into shape, using a specialized jig and crank he had created. To his surprise, it worked nearly perfectly. Sreetharan is a pianist, and he remembers thinking as the bee emerged that there was something symphonic about the process. “It has so many different parts that are all basically in harmony,” he says. “Nothing in it is still. Everything happens together in such an ordered and controlled way.” His latest RoboBees can be popped into shape in less than a tenth of a second—he had to slow the process to make a video of it. Their predecessors took a week to make by hand.
For many researchers the true measure of folding’s potential is the profusion of ways nature uses it. One of Wood’s colleagues and collaborators is L. Mahadevan, a Harvard mathematician who studies the fold algorithms of insect wings, leaves, and flowers. Also at the Wyss, a biophysicist named Shawn Douglas, working with immunologist Ido Bachelet, has built something called a DNA origami nanorobot, a drug-delivery device one-ten-thousandth of the width of the period at the end of this sentence. The bot has shown the ability, at least in petri dishes, to identify cancerous cells and release a payload of antibodies that kills them while leaving healthy cells untouched.
Douglas and Bachelet built their smart bombs using DNA origami, which takes advantage of the way DNA base pairs—the teeth in the zipper of the double helix—bond to each other. The method involves mixing single strands of DNA to form a three-dimensional structural lattice. By determining the base pairs’ order, DNA origami designers can determine where the strands bond and thus the three-dimensional form the lattice folds itself into. It’s not really origami; it’s more like the world’s smallest, most complicated balloon animal. Researchers elsewhere are working on the puzzle of how proteins fold. The building blocks of human cells, proteins are strands of amino acids—they are extruded, spaghetti-like, from cellular machines called ribosomes. Biologists still don’t fully understand what determines the intricate shapes that the long molecules snap into once they’re completed. It’s a question with huge ramifications—Alzheimer’s, mad cow, and various cancers are thought to be caused by protein misfolding. One particularly promising approach to understanding protein folding is a website called Foldit, created by David Baker, a biochemist at the University of Washington. It’s a game—anyone can go to the website and play—that awards points for finding the most likely folding pattern for a protein. Certain players have proven to have a real knack for it, and their cumulative efforts have solved problems that have long stumped biochemists.
The goal of researchers such as Baker isn’t just understanding how protein folding works and how it can go awry but also designing entirely new proteins. The forms proteins take determine their function—the corkscrew shape of actin is the mechanism by which muscles contract, the long, clingy arms of fibrin molecules form blood clots. Creating proteins that fold into new shapes could lead to compounds with new abilities: pharmaceuticals, chemical catalysts, molecules that take carbon dioxide out of the air or cause toxic compounds to break down. Baker has developed a protein that seems to block H1N1 flu infection, and Foldit players are at work on similar proteins for other flu viruses. As Baker describes it, what he and the Foldit players are doing is essentially protein origami. “Living systems have all these different things that they can do and all of these different chemical reactions that they can catalyze, but those are just the chemical reactions that were of interest during the evolution of life,” he says. “We in our modern world have a whole bunch of problems that were never encountered during evolution, so we’d like to be able to make new proteins that solve those problems.”
Demaine has also studied protein folding. Along with advanced geometry, he’s using some of the same assumptions behind Foldit. “If we can find efficient, nice folding algorithms for proteins,” he says, “maybe nature is following those.” What excites him at the moment, though, is something called curved-crease folding, a method that dates to the 1920s and the artists and designers of the Bauhaus. Curving folds, it turns out, have a particular power: a simple pattern can create a menagerie of intricate three-dimensional shapes and can change the qualities of a piece of paper, giving it the ability to stretch and drape in new ways. Demaine is curious why that is. “There’s very little theory on curved-crease origami,” he says. He’s not sure whether anything useful will emerge from the work, and as a mathematician that’s not his concern. His father is now an artist-in-residence and visiting scientist at MIT, and together the two have created a series of curved-crease sculptures. Some are in the permanent collection of the Smithsonian American Art Museum, others were on view earlier this spring at a Manhattan gallery. They look like some sort of mutant mollusk rendered by M.C. Escher. They seem, at the same time, both impossible and perfectly natural.
This article firstly appeared on bloomberg.com On May 4, 2012, written by Drake Bennet. Images credit Leonard Greco for Bloomberg Businessweek. Note: Materials may be edited for content and length.
#Biology #Robotics